Dr. Piña-Crespo earned a PhD in Pharmacology from University College London (UCL), England under the supervision of Profs. Alasdair Gibb & David Colquhoun FRS. He completed postdoctoral training as a Pew Fellow/Research Associate with Prof. Steve Heinemann in the Molecular Neurobiology Laboratory at The Salk Institute, La Jolla, California. Dr. Piña-Crespo has held faculty positions as Instructor and Assistant Professor at Universidad Centroccidental, Venezuela and as Lecturer in the Biology Department at the University of San Diego, California.
Education and Training
Postdoctoral training (Pew Fellow/Research Associate) The Salk Institute, California
PhD in Pharmacology University College London (University of London), England
Veterinarian (DVM) Universidad Centroccidental Lisandro Alvarado, Venezuela
Honors and Recognition
Pew Fellow in the Biomedical Sciences
Related Disease
Aging-Related Diseases, Alzheimer’s Disease, Brain Injury, Epilepsy, Molecular Biology, Nervous System Injury, Neurodegenerative and Neuromuscular Diseases, Neurological and Psychiatric Disorders, Parkinson’s Disease, Stroke, Traumatic Injury
Phenomena or Processes
Aging, Apoptosis and Cell Death, Calcium Signaling, Cell Biology, Cell Signaling, Cell Surface Receptors, Development of Neuronal Circuits, Disease Therapies, Neurobiology, Neurogenesis, Neuron-Glia Interactions in Myelin, Neurotransmitters, Synapse Function, Synaptic Transmission
Anatomical Systems and Sites
Brain, General Cell Biology, Nervous System
Research Models
Cultured Cell Lines, Human Cell Lines, Human Embryonic Stem Cells, Mouse, Mouse Cell Lines, Primary Cells, Primary Human Cells, Rat, Vertebrates, Xenopus
Techniques and Technologies
Biophysics, Biophysiology, Calcium Imaging, Cellular and Molecular Imaging, Electrophysiology, Fluorescence Microscopy, Ion Channels, Live Cell Imaging, Mouse Behavioral Analysis, Pharmacology, Transplantation
Working on basic neuroscience discovery research. I use cellular and animal models of neurodegeneration to identify basic disease-causing mechanisms and disease-relevant targets involved in abnormal neuron-glia signaling, synapse failure, neuronal network dysfunction and neuronal loss in age-related neurodegenerative diseases; including Alzheimer’s and Parkinson’s disease. Extensive hands-on experience working and managing projects that require a strong background in in-vitro, ex-vivo and in-vivo neuroscience, pharmacology and electrophysiology.
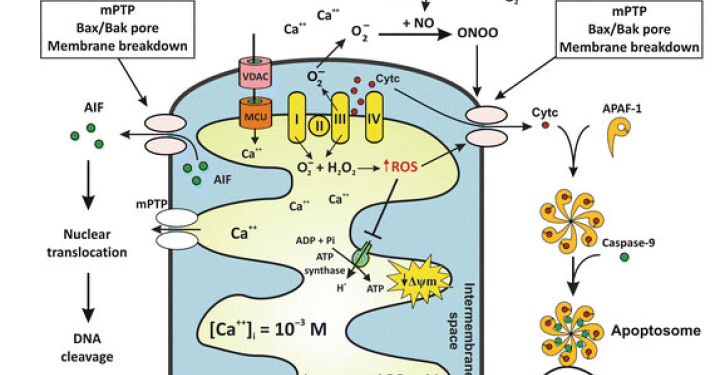