Funds will support Alicia Llorente Lope and Ambroise Manceau who study breast and pancreatic cancer
Alicia Llorente Lope, PhD, and Ambroise Manceau, PhD, were awarded 2024 Postdoctoral Fellowships from the American Cancer Society (ACS). These prestigious awards provide more than $65,000 per year for up to three years to support early career scientists studying cancer.
“I was so excited when I heard the news,” said Llorente. “It is a privilege to have this award, and it feels very validating to know that someone saw enough potential in my research to deem it worthy of funding.”
Tackling treatment-resistant breast cancer
Llorente joined the lab of Brooke Emerling, PhD, director of the Cancer Metabolism and Microenvironment Program at Sanford Burnham Prebys, nearly three years ago after beginning her breast cancer research career as a doctoral student.
“I was first interested in breast cancer because my grandmother died of the disease, and I wanted to contribute to finding new therapeutic opportunities for cancer patients,” said Llorente. Llorente’s ACS-funded research project focuses on HER2-positive (HER2+) breast cancer.
Roughly one in five breast cancer tumors have elevated levels of the HER2 protein. While these tumors tend to grow quickly, drugs targeting the HER2 protein are usually effective at first. However, HER2+ tumors often are able to adapt and develop resistance to these drugs over time, leaving patients with few if any remaining treatments options.
Llorente has found evidence that a form of the protein phosphatidylinositol-5-phosphate 4-kinase (PI5P4K) plays a role in breast cancer tumors becoming resistant to HER2 drugs.
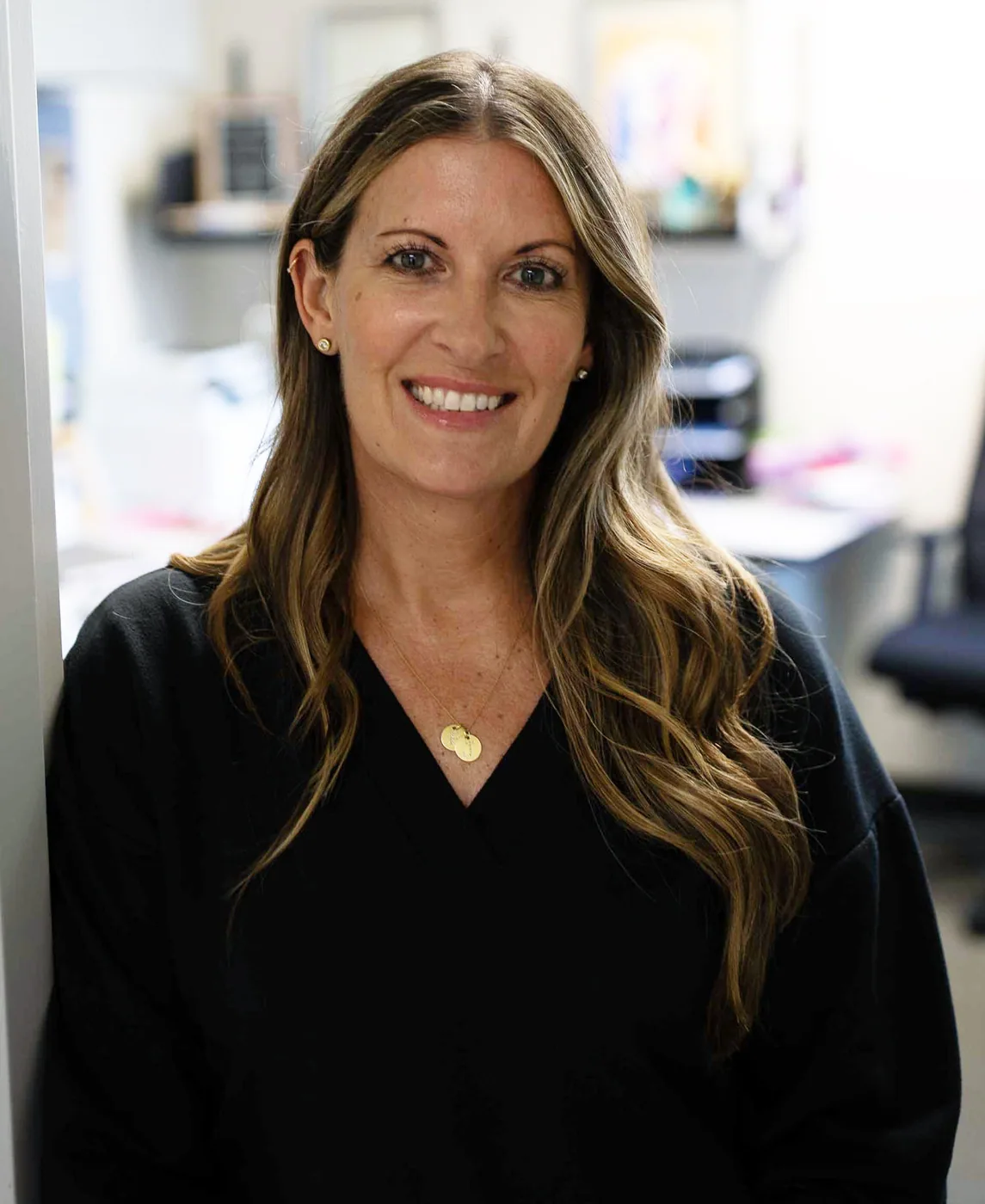
Brooke Emerling, PhD
“We’ve revealed a strong connection between elevated levels of PI5P4K gamma and reduced survival rates in patients with HER2+ breast cancer,” explained Llorente. “I plan to explore whether targeting both HER2 and PI5P4K gamma in breast cancer cells may provide a path to overcoming treatment resistance.” Llorente also will study the functions of PI5P4K gamma in breast cancer cells to see why these cells cease to respond to HER2-targeting drugs.
“I am incredibly proud of Alicia for spearheading this groundbreaking project targeting the lipid kinase PI5P4K gamma,” said Emerling. “Her insightful analysis of breast cancer datasets, which uncovered a correlation between elevated expression of PI5P4K gamma and worse outcomes in HER2+ patients, has set the stage for vital research aimed at overcoming the significant challenge of resistance to targeted therapies in HER2+ tumors.”
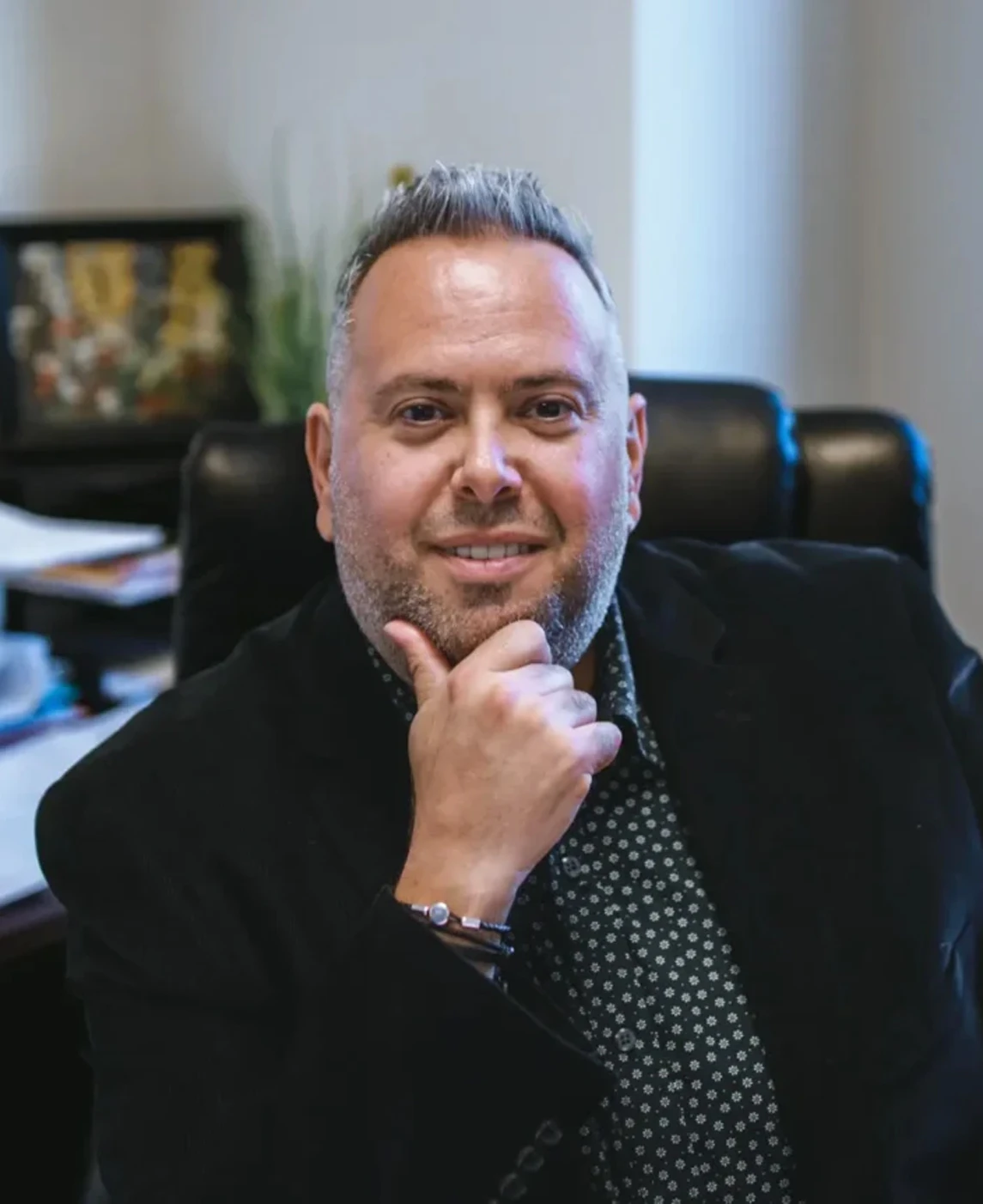
Cosimo Commisso, PhD
Powering down pancreatic cancer
Manceau is in the second year of his postdoctoral training in the lab of Cosimo Commisso, PhD, interim director and deputy director of the institute’s NCI-Designated Cancer Center. During his doctoral program, Manceau studied how abnormal cells die in a programmed series of steps called apoptosis, a process known to go awry in cancer and neurodegenerative diseases.
“It began as a basic science project about the molecular processes around cell death, and over time it led to possible therapeutic implications,” said Manceau. “I learned that I like to study fundamental biology and then try to find an application for it, and I saw in the Commisso lab an opportunity to do just that in pancreatic cancer.”
Manceau’s fellowship project focuses on pancreatic ductal adenocarcinoma (PDAC) — the most common form of pancreatic cancer with only a 13% five-year survival rate — and its ravenous pursuit of energy. Because of PDAC cells’ constant need for fuel to sustain their rampant growth, they adapt by reshaping the surface of their cells to snatch extra nutrients from the jelly-like substance between cells.
Commisso and others have shown that cutting off the extra power supplied by this process — known as macropinocytosis — reduces tumor growth. Manceau has studied the contents taken by contorted pancreatic cell surfaces in pockets called macropinosomes. By analyzing every single protein in this scooped goop, he found that calcium transporter proteins present in macropinosomes also are required for macropinocytosis.
“During the fellowship, I will work to understand how these transporter proteins affect macropinocytosis,” said Manceau. “These proteins have never been targeted before in pancreatic cancer, so our long-term goal is to use this strategy to cut the nutrient supply to tumors and see if we can inhibit tumor growth.”
“By disrupting the cancer cells’ ability to feed themselves through macropinocytosis, we can potentially starve tumors and inhibit their growth,” added Commisso. “Ambroise’s research aims to target key proteins involved in this process, opening up new possibilities for treatments that could significantly improve outcomes for patients battling pancreatic cancer.”